Excitation and Inhibition: The Yin and Yang of the Brain
Excitation and Inhibition: The Yin and Yang of the Brain
Beautiful complexity arises from the balance of two opposing forces.
Source: Kayleen Schreiber, Knowing Neurons
The balance between neural excitation and neural inhibition is crucial to healthy cognition and behavior. A brain dominated by glutamate would only be capable of exciting itself in repeated bursts of activity, similar to an epileptic seizure. Conversely, a brain dominated by GABA would only be capable of quiet whispers of activity, with little synchronization necessary for meaningful communication between brain areas. Healthy brain activity thrives in the middle area between these two extremes, where a balance between excitation and inhibition generates complex patterns of activity. Thus, a seemingly simple nervous system formed with only glutamate and GABA nonetheless results in highly complex activity.
Similarly, a seemingly simple mixture of chemicals in a Petri dish can give rise to highly complex chemical reaction patterns, such as oscillating spiral waves, when a chemical that excites the reaction and a chemical that inhibits the reaction are both present. This general type of reaction, called the Belousov–Zhabotinsky reaction, has even been studied as a model for how neural networks process information, since the reaction’s complexity is governed by similar principles.
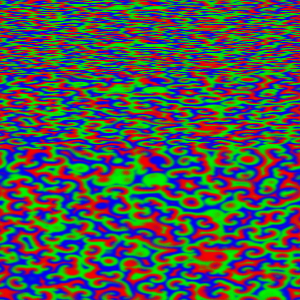
Simulation of the Belousov–Zhabotinsky reaction
Source: Wikimedia Commons user Simpsons Contributor
How does the synergy between excitation and inhibition work? Both excitation and inhibition, acting alone, attract the brain toward distinct patterns of relatively simple activity. The balance of both creates a critical state, like the boundary between a gas and a liquid. Outside the brain, many critical states are unstable, like a pencil that is balanced vertically on its tip but falls over after any further change in its position. Yet surprisingly, critical states in the brain are often self-maintained and robust to further changes. For instance, after synaptic input to a neural network has generated a critical state, further synaptic input maintains the critical state rather than pushing the network into a simple, stable pattern. For this reason, the phenomenon is called self-organized criticality, or SOC, a term for the concept developed by physicists Per Bak, Chao Tang, and Kurt Wiesenfeld of the Brookhaven National Laboratory in New York.
SOC is thought to be important for brain function because it allows the brain a certain degree of flexibility. Just as a critical substance might flexibly switch between a gas and a liquid state, SOC might allow the brain to visit many different activity states. Wherever SOC is observed in nature, it seems to produce complex activity across many temporal and spatial scales as a result of a slow process that builds energy and a fast process that dissipates energy. This complexity can be described by a pattern called a scale-free distribution. Unlike the normal distribution or “bell curve” we know from statistics class, a scale-free distribution has no mean or average.
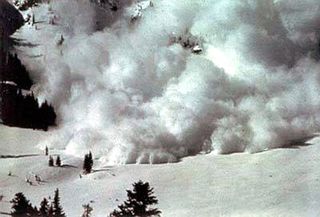
Criticality in the brain can be understood by imagining avalanches forming on a sandpile or mountain.
Source: Wikimedia Commons user Ben-Zin
The two competing processes in this example are the slow process of adding sand, which builds energy, and the fast process resulting from the force of gravity overcoming the force friction, which dissipates energy. Perhaps this example feels far removed from the brain. But the slow process of adding sand is actually analogous to adding excitatory synaptic input in a neural network. Similarly, the fast process of gravity overcoming friction is analogous to neural excitation overcoming neural inhibition and triggering bursts of firing—neuronal avalanches. Sandpile avalanches follow the same scale-free distribution observed in electrical brain recordings: Activity is observed at all scales and frequencies, a result of delicate E/I balance.
Source: Kayleen Schreiber, Knowing Neurons
Because electrical brain activity can easily be observed by placing electrodes on the scalp (EEG), it is possible for researchers and clinicians to infer E/I balance without directly probing cells in the brain. For example, epileptiform discharges—bursts of disruptive excitement—are clear signatures of a high E/I ratio. These discharges may indicate that the brain has been pushed past criticality to a supercritical state. Though traditionally associated with epilepsy, epileptiform discharges may also occur in the EEGs of patients who have never had a single seizure before. An emerging concept of epilepsy spectrum disorders seeks to frame mental illnesses, such as panic disorder, in the same context as epilepsy. Dr. Nash N. Boutros at the University of Missouri, Kansas City is exploring epileptiform discharges in patients with panic attacks as possible indicators of the same high E/I ratio that causes epilepsy. If panic disorders and epilepsy share a common cause, they might both be treatable with antiepileptic drugs. While such drugs generally treat seizures, they are believed to decrease neuronal excitability and have also been approved by the FDA to treat bipolar disorder, a psychiatric disorder where patients experience states of both elevated and lowered mood.
In the near future, drugs that alter neuronal excitability may show promise in guiding the diseased brain towards E/I balance. Indeed, just as many spiritual practices advocate for maintaining an “inner balance,” a physical balance between opposing forces appears central to maintaining a healthy brain. The synergy between opposites observed in the brain reminds us that complexity requires a balance. While empirical evidence has shown that brain size or brain mass is not the best measure of brain fitness, E/I balance might instead contend for that title. One day, a checkup at the doctor’s office might not just involve taking your pulse, height, and weight, but also an EEG reading of your E/I ratio